Quorum sensing: Difference between revisions
imported>David Tribe No edit summary |
imported>Meg Taylor m (grammar: gram -> Gram) |
||
(48 intermediate revisions by 6 users not shown) | |||
Line 1: | Line 1: | ||
{{subpages}} | |||
{{main|Microbial cell and population biology}} | |||
'''Quorum sensing''' is the ability of populations of [[bacterium|bacteria]] to communicate and coordinate their behavior via inter-cellular and inter-species signaling [[molecule]]s. | |||
A general name for quorum sensing signaling compounds is '''autoinducer''', referring to their ability to trigger gene expression in the cells (autoinduction) which belong to the same species that produces the compound. '''[[Pheromone]]''' is another term with a very similar meaning to '''autoinducer'''. | |||
In Gram-negative bacteria, at least two main classes of autoinducer have been defined, namely AI-1 and AI-2. AI-1s are various forms of '''acyl [[Homoserine Lactone|homoserine lactone]]s''' (AHLs). AHLs are synthesized from acyl carrier proteins by LuxI-type AHL synthases. The enzyme producing AI-2 is called called LuxS, which forms the common AH-2 precursor molecule '''4,5-dihydroxy-2,3-pentanedione (DPD)''' that undergoes a variety of spontaneous rearrangements which generate different AI-2 compounds used by different species. | |||
In Gram-positive bacteria such as Bacillus and Enterococcus, autoinducers are usually '''short peptides'''<ref>Camilli A and Bassler BL (2006). Bacterial small-molecule signaling pathways. Science 311: 1113-1116.</ref>. | |||
==General biological function of quorum sensing== | |||
A major function of quorum sensing is to coordinate certain behaviour or actions between bacteria of the same kind, depending on their number. The use of the word quorum is an allusion to the attendance numbers required for valid business meetings that legally require a "quorum". | |||
An example of bacterial coordination is when opportunistic bacteria, such as ''[[Pseudomonas aeruginosa]]'' can grow within a host without harming it, until they reach a certain concentration. Then at a certain cell density (the quorum) they can channel their activities into activities (such as specialised [[biofilm]] formation and immune system suppression) where the presence of large number of cooperating bacteria may successfully overwhelm the large host <ref>Pradeep K. Singh, Amy L. Schaefer, Matthew R. Parsek,Thomas O. Moningerk, Michael J. Welsh, & E. P. Greenberg. (2000) Quorum-sensing signals indicate that cystic fibrosis lungs are infected with bacterial biofilms NATURE VOL 407 12 OCTOBER 2000 page 762-762.</ref>. | |||
The | An autoinducer signal of one species can be used as a cue by another species. The opportunistic pathogen ''P. aeruginosa'' in cystic fibrosis may respond to chemical clues from other throat flora . In this case, the Gram-positive throat flora produces significant levels of AI-2, while ''P. aeruginosa'' itself does not produce AI-2. But by being able to respond to AI-2, ''P. aeruginosa'' can be said to be using AI-2 as a "cue", rather than for cooperation. <ref> Keller, Laurent and Surette, Michael G. (2006) Communication in bacteria: an ecological and evolutionary perspective. Nature Reviews Microbiology 4, 249-258 (April 2006) | doi:10.1038/nrmicro1383 </ref> | ||
It is hoped that the therapeutic useful enzymatic degradation of the signalling molecules might be used in the future prevent the formation of bacterial biofilms and possibly weaken established biofilms. Disrupting the signalling process in this way is called ''quorum quenching''. | |||
==Role of quorum sensing in specific organisms== | |||
Quorum sensing has been implicated in many different microorganisms as a mechanism for triggering of a wide range of complex or highly evolved responses whose effectiveness depends on large numbers of cells with the same capabilities working together in a particular location. These include <ref> Camilli A and Bassler BL (2006). Bacterial small-molecule signaling pathways. Science 311: 1113-1116. </ref> <ref>Waters CM and Bassler BL (2005). Quorum sensing: cell-to-cell communication in bacteria. Annu Rev Cell Dev Biol 21: 319-346. </ref>: | |||
* [[Bioluminescence]] by Vibrio bacteria, discovered in the 1970s | |||
* Mating ability of the Gram-positive coccus ''Enterococcus faecalis'', Clewell, 1975 | |||
* Cytolysin (virulence factor) production by Enterococcus | |||
* Antibiotic production by various Streptomyces and Erwinia bacteria | |||
* Fruiting body production by Myxococcus bacteria<ref>Shimkets, L. J. (1999) Intercellular signaling during fruiting-body development of Myxococcus xanthus. Annu. Rev. Microbiol. 53, pages 525–549.</ref> <ref>Kruse, T. , Lobedanz, S. , Berthelsen, N. M. & Sogaard-Andersen, L. (2001) C-signal: a cell surface-associated morphogen that induces and co-ordinates multicellular fruiting body morphogenesis and sporulation in Myxococcus xanthus. Mol. Microbiol. 40, pages 156–168 .</ref> | |||
* Virulence, biofilm formation by ''[[Pseudomonas aeruginosa]]'' | |||
* Biofilm formation ability coded by F factor in ''Escherichia coli'' | |||
* Virulence in ''[[Vibrio cholerae]]'' | |||
* DNA injection into plants by ''[[Agrobacterium tumefaciens]]]'' | |||
* Erwinia bacteria's virulence for plants <ref>Tom Burr, Anne M. L. Barnard, Mark J. Corbett, Clare L. Pemberton, Natalie J. L. Simpson and George P. C. Salmond (2006) Identification of the central quorum sensing regulator of virulence in the enteric phytopathogen, Erwinia carotovora: the VirR repressor. Molecular Microbiology Volume 59 Issue 1 Page 113 - January 2006 doi:10.1111/j.1365-2958.2005.04939.x Volume 59 Issue 1</ref> | |||
* DNA uptake ([[transformation]]) competence in ''Streptococcus pneumoniae'' | |||
* Competence for DNA [[transformation]] , spore formation in Bacillus <ref>Solomon, J. M. , Lazazzera, B. A. & Grossman, A. D.(1996) Purification and characterization of an extracellular peptide factor that affects two different developmental pathways in Bacillus subtilis. Genes Dev. 10, pages 2014–2024.</ref> | |||
* ''[[Staphylococcus aureus]]'' virulence <ref>Shompole, S. et al.(2003) Biphasic intracellular expression of Staphylococcus aureus virulence factors and evidence for Agr-mediated diffusion sensing. Mol. Microbiol. 49, 919–927 </ref> | |||
The first [[organism]]s in which quorum sensing was observed were [[Myxobacteria]] and [[Streptomyces]] species. However, the most well known (and beautiful) example is the regulation of light production in ''[[Vibrio fischeri]]'', a bioluminiscent bacterium that lives as a [[symbiosis|symbiont]] in the light-producing organ of the Hawaiian [[bobtail squid]]. When ''V. fischeri'' cells are free-living, the autoinducer is at low concentration and thus cells do not luminesce. In the light organ of the squid ([[photophore]]), they are highly concentrated (about 10<sup>11</sup> cells/ml) and transcription of [[luciferase]] is induced, leading to [[bioluminescence]]. | |||
Processes possibly regulated or partially regulated by AI-2-mediated quorum sensing in ''[[E. coli]]'' include [[cell division]]. In other species such as ''[[Pseudomonas aeruginosa]]'' quorum-related processes include biofilm development, exopolysaccharide production, and cell aggregation. AI-2 was found to increase expression of sdiA, a transcriptional regulator of promoters which promote ftsQ, part of the ftsQAZ operon essential for cell division. | |||
''[[Streptococcus pneumoniae]]'' uses quorum sensing to become [[competent cells|competent]] for DNA uptake natural gene [[transformation]]. | |||
[[ | |||
==Methods and mechanisms of quorum sensing== | ==Methods and mechanisms of quorum sensing== | ||
[[Image:Quorumsensing_VIBRIO_CHOLERAE.jpg|right|frame|Figure 1. Quorum-Sensing Circuit of the Bacterial Pathogen ''Vibrio cholerae'' Red arrows indicate phosphoryl-group transfer [1]. (Figure: Matthew B. Neiditch, Princeton University, Princeton, New Jersey, United States). [http://biology.plosjournals.org/perlserv/?request=get-document&doi=10.1371%2Fjournal.pbio.0040299 Cooperation among Microorganisms Ned S. Wingreen, Simon A. Levin PLoS Biol 4(9): e299. DOI:10.1371/journal.pbio.0040299 Published: September 12, 2006] ]] | |||
Bacteria that use quorum sensing produce and secrete certain signaling [[chemical compound|compounds]] (called ''autoinducers'' or ''[[pheromone]]s''), one example of which are ''N''-acyl [[Homoserine Lactone|homoserine lactone]]s (AHL). These bacteria also have a [[receptor (biochemistry)|receptor]] that can specifically detect the AHL ([[inducer]]). When the inducer binds the receptor, it activates [[Transcription (genetics)|transcription]] of certain [[gene]]s, including those for inducer synthesis. There is a low likelihood of a bacterium detecting its own secreted AHL. | Bacteria that use quorum sensing produce and secrete certain signaling [[chemical compound|compounds]] (called ''autoinducers'' or ''[[pheromone]]s''), one example of which are ''N''-acyl [[Homoserine Lactone|homoserine lactone]]s (AHL). These bacteria also have a [[receptor (biochemistry)|receptor]] that can specifically detect the AHL ([[inducer]]). When the inducer binds the receptor, it activates [[Transcription (genetics)|transcription]] of certain [[gene]]s, including those for inducer synthesis. There is a low likelihood of a bacterium detecting its own secreted AHL. | ||
Line 103: | Line 56: | ||
In ''[[Escherichia coli]]'', AI-2 is produced by the lsr [[operon]], encoding an [[ATP-binding cassette transporter genes|ABC transporter]] which imports AI-2 into the cells during the early stationary (latent) phase of growth. AI-2 is then phosphorylated by lsrK and the newly produced phospho-AI-2 can either be internalized or used to suppress lsrR, an inhibitor of the lsr operon (thereby activating the operon). The lsr operon is also thought to be inhibited by [[dihydroxyacetone phosphate]] (DHAP) through its competitive binding to lsrR. [[Glyceraldehyde 3-phosphate]] has also been shown to inhibit the lsr operon through cAMP-CAPK-mediated inhibition. This explains why when grown with glucose ''E. coli'' will lose the ability to internalize AI-2 (because of catabolite repression). When grown normally, AI-2 presence is transient. | In ''[[Escherichia coli]]'', AI-2 is produced by the lsr [[operon]], encoding an [[ATP-binding cassette transporter genes|ABC transporter]] which imports AI-2 into the cells during the early stationary (latent) phase of growth. AI-2 is then phosphorylated by lsrK and the newly produced phospho-AI-2 can either be internalized or used to suppress lsrR, an inhibitor of the lsr operon (thereby activating the operon). The lsr operon is also thought to be inhibited by [[dihydroxyacetone phosphate]] (DHAP) through its competitive binding to lsrR. [[Glyceraldehyde 3-phosphate]] has also been shown to inhibit the lsr operon through cAMP-CAPK-mediated inhibition. This explains why when grown with glucose ''E. coli'' will lose the ability to internalize AI-2 (because of catabolite repression). When grown normally, AI-2 presence is transient. | ||
A first [[X-ray structure]] of a receptor (LuxP) was discovered in ''[[Vibrio harveyi]]'' in 2002, together with its inducer (''AI-2''), which is one of the few [[biomolecules]] containing [[boron]] (''Nature'' '''415''', 545ff [http://www.nsls.bnl.gov/newsroom/science/2002/pdfs/2002-09-Hughson.pdf PDF]). Autoinducer-2 is conserved among many bacterial species, including ''Escherichia coli'', an enteric bacterium and model organism for Gram negative bacteria. Autoinducer-2 appears to be used for interspecies communication because of this conservation. | A first [[X-ray structure]] of a receptor (LuxP) was discovered in ''[[Vibrio harveyi]]'' in 2002, together with its inducer (''AI-2''), which is one of the few [[biomolecules]] containing [[boron]] (''Nature'' '''415''', 545ff [http://www.nsls.bnl.gov/newsroom/science/2002/pdfs/2002-09-Hughson.pdf PDF]). Autoinducer-2 is conserved among many bacterial species, including ''Escherichia coli'', an enteric bacterium and model organism for Gram-negative bacteria. Autoinducer-2 appears to be used for interspecies communication because of this conservation. | ||
==References== | ==References== | ||
===Citations=== | |||
<references/> | <references/> | ||
===Further reading=== | ===Further reading=== | ||
* Banin, E., M. L. Vasil, and E. P. Greenberg (2005). Iron and Pseudomonas aeruginosa biofilm formation. Proc Natl Acad Sci U S A 102: 11076-81. | * Banin, E., M. L. Vasil, and E. P. Greenberg (2005). Iron and Pseudomonas aeruginosa biofilm formation. Proc Natl Acad Sci U S A 102: 11076-81. | ||
* Bassler BL (2006). Cell-to-cell communication in bacteria: a chemical discourse. Harvey Lect. 2004-2005 100: 123-142. | * Bassler BL (2006). Cell-to-cell communication in bacteria: a chemical discourse. Harvey Lect. 2004-2005 100: 123-142. | ||
* Bassler BL and Losick R (2006). Bacterially speaking. Cell 125: 237-246. | * Bassler BL and Losick R (2006). Bacterially speaking. Cell 125: 237-246. | ||
Camilli A and Bassler BL (2006). Bacterial small-molecule signaling pathways. Science 311: 1113-1116. | * Camilli A and Bassler BL (2006). Bacterial small-molecule signaling pathways. Science 311: 1113-1116. | ||
* Kolter, R. and E. P. Greenberg (2006). Microbial sciences: the superficial life of microbes. Nature 441: 300-2. | * Kolter, R. and E. P. Greenberg (2006). Microbial sciences: the superficial life of microbes. Nature 441: 300-2. | ||
* Waters CM and Bassler BL (2005). Quorum sensing: cell-to-cell communication in bacteria. Annu Rev Cell Dev Biol 21: 319-346. | * Waters CM and Bassler BL (2005). Quorum sensing: cell-to-cell communication in bacteria. Annu Rev Cell Dev Biol 21: 319-346. | ||
* Waters CM, Bassler BL (2006). The Vibrio harveyi quorum-sensing system uses shared regulatory components to discriminate between multiple autoinducers. Genes Dev 20: 2754-2767. | * Waters CM, Bassler BL (2006). The ''Vibrio harveyi'' quorum-sensing system uses shared regulatory components to discriminate between multiple autoinducers. Genes Dev 20: 2754-2767. | ||
* Xavier KB and Bassler BL (2005). Regulation of uptake and processing of the quorum-sensing autoinducer AI-2 in Escherichia coli. J Bacteriol 187: 238-248. | * Xavier KB and Bassler BL (2005). Regulation of uptake and processing of the quorum-sensing autoinducer AI-2 in ''Escherichia coli''. J Bacteriol 187: 238-248. | ||
* Xavier KB and Bassler BL (2005). Interference with AI-2-mediated bacterial cell-cell communication. Nature 437: 750-753. | * Xavier KB and Bassler BL (2005). Interference with AI-2-mediated bacterial cell-cell communication. Nature 437: 750-753. | ||
===External links=== | ===External links=== | ||
* [http://www.nottingham.ac.uk/quorum The Quorum Sensing Website] | * [http://www.nottingham.ac.uk/quorum The Quorum Sensing Website] | ||
* [http://www.genesdev.org/cgi/ijlink?linkType=FULL&journalCode=iai&resid=68/9/4839 Bacterial quorum sensing in pathogenic relationships. (Infect. Immunol. 68: 4839-4849) Free Full Text 2000] | * [http://www.genesdev.org/cgi/ijlink?linkType=FULL&journalCode=iai&resid=68/9/4839 Bacterial quorum sensing in pathogenic relationships. (Infect. Immunol. 68: 4839-4849) Free Full Text 2000] | ||
* [http://www.hhmi.org/research/investigators/bassler.html Cell-to-Cell Communication in Bacteria] | * [http://www.hhmi.org/research/investigators/bassler.html Cell-to-Cell Communication in Bacteria] | ||
*[http://www.molbio2.princeton.edu/index.php?option=content&task=view&id=27 Department of Molecular Biology, Princeton University] | *[http://www.molbio2.princeton.edu/index.php?option=content&task=view&id=27 Department of Molecular Biology, Princeton University]. | ||
Revision as of 02:20, 16 February 2010

Quorum sensing is the ability of populations of bacteria to communicate and coordinate their behavior via inter-cellular and inter-species signaling molecules.
A general name for quorum sensing signaling compounds is autoinducer, referring to their ability to trigger gene expression in the cells (autoinduction) which belong to the same species that produces the compound. Pheromone is another term with a very similar meaning to autoinducer.
In Gram-negative bacteria, at least two main classes of autoinducer have been defined, namely AI-1 and AI-2. AI-1s are various forms of acyl homoserine lactones (AHLs). AHLs are synthesized from acyl carrier proteins by LuxI-type AHL synthases. The enzyme producing AI-2 is called called LuxS, which forms the common AH-2 precursor molecule 4,5-dihydroxy-2,3-pentanedione (DPD) that undergoes a variety of spontaneous rearrangements which generate different AI-2 compounds used by different species.
In Gram-positive bacteria such as Bacillus and Enterococcus, autoinducers are usually short peptides[1].
General biological function of quorum sensing
A major function of quorum sensing is to coordinate certain behaviour or actions between bacteria of the same kind, depending on their number. The use of the word quorum is an allusion to the attendance numbers required for valid business meetings that legally require a "quorum".
An example of bacterial coordination is when opportunistic bacteria, such as Pseudomonas aeruginosa can grow within a host without harming it, until they reach a certain concentration. Then at a certain cell density (the quorum) they can channel their activities into activities (such as specialised biofilm formation and immune system suppression) where the presence of large number of cooperating bacteria may successfully overwhelm the large host [2].
An autoinducer signal of one species can be used as a cue by another species. The opportunistic pathogen P. aeruginosa in cystic fibrosis may respond to chemical clues from other throat flora . In this case, the Gram-positive throat flora produces significant levels of AI-2, while P. aeruginosa itself does not produce AI-2. But by being able to respond to AI-2, P. aeruginosa can be said to be using AI-2 as a "cue", rather than for cooperation. [3]
It is hoped that the therapeutic useful enzymatic degradation of the signalling molecules might be used in the future prevent the formation of bacterial biofilms and possibly weaken established biofilms. Disrupting the signalling process in this way is called quorum quenching.
Role of quorum sensing in specific organisms
Quorum sensing has been implicated in many different microorganisms as a mechanism for triggering of a wide range of complex or highly evolved responses whose effectiveness depends on large numbers of cells with the same capabilities working together in a particular location. These include [4] [5]:
- Bioluminescence by Vibrio bacteria, discovered in the 1970s
- Mating ability of the Gram-positive coccus Enterococcus faecalis, Clewell, 1975
- Cytolysin (virulence factor) production by Enterococcus
- Antibiotic production by various Streptomyces and Erwinia bacteria
- Fruiting body production by Myxococcus bacteria[6] [7]
- Virulence, biofilm formation by Pseudomonas aeruginosa
- Biofilm formation ability coded by F factor in Escherichia coli
- Virulence in Vibrio cholerae
- DNA injection into plants by Agrobacterium tumefaciens]
- Erwinia bacteria's virulence for plants [8]
- DNA uptake (transformation) competence in Streptococcus pneumoniae
- Competence for DNA transformation , spore formation in Bacillus [9]
- Staphylococcus aureus virulence [10]
The first organisms in which quorum sensing was observed were Myxobacteria and Streptomyces species. However, the most well known (and beautiful) example is the regulation of light production in Vibrio fischeri, a bioluminiscent bacterium that lives as a symbiont in the light-producing organ of the Hawaiian bobtail squid. When V. fischeri cells are free-living, the autoinducer is at low concentration and thus cells do not luminesce. In the light organ of the squid (photophore), they are highly concentrated (about 1011 cells/ml) and transcription of luciferase is induced, leading to bioluminescence.
Processes possibly regulated or partially regulated by AI-2-mediated quorum sensing in E. coli include cell division. In other species such as Pseudomonas aeruginosa quorum-related processes include biofilm development, exopolysaccharide production, and cell aggregation. AI-2 was found to increase expression of sdiA, a transcriptional regulator of promoters which promote ftsQ, part of the ftsQAZ operon essential for cell division.
Streptococcus pneumoniae uses quorum sensing to become competent for DNA uptake natural gene transformation.
Methods and mechanisms of quorum sensing
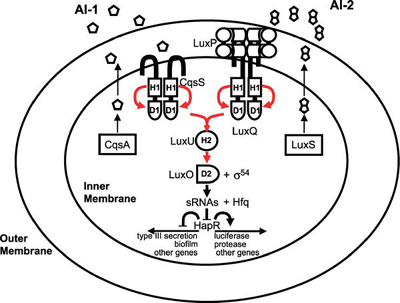
Bacteria that use quorum sensing produce and secrete certain signaling compounds (called autoinducers or pheromones), one example of which are N-acyl homoserine lactones (AHL). These bacteria also have a receptor that can specifically detect the AHL (inducer). When the inducer binds the receptor, it activates transcription of certain genes, including those for inducer synthesis. There is a low likelihood of a bacterium detecting its own secreted AHL.
When only a few other bacteria of the same kind are in the vicinity, diffusion reduces the concentration of the inducer in the surrounding medium to almost zero, so the bacteria produce little inducer. With many bacteria of the same kind, the concentration of the inducer passes a threshold, whereupon more inducer is synthesised. This forms a positive feedback loop, and the receptor becomes fully activated. This induces the up regulation of other specific genes, such as luciferase in V. fishcheri. This is useful since a single V. fischeri bacterium that is luminescent would have no evolutionary advantage and would be wasting energy.
In Escherichia coli, AI-2 is produced by the lsr operon, encoding an ABC transporter which imports AI-2 into the cells during the early stationary (latent) phase of growth. AI-2 is then phosphorylated by lsrK and the newly produced phospho-AI-2 can either be internalized or used to suppress lsrR, an inhibitor of the lsr operon (thereby activating the operon). The lsr operon is also thought to be inhibited by dihydroxyacetone phosphate (DHAP) through its competitive binding to lsrR. Glyceraldehyde 3-phosphate has also been shown to inhibit the lsr operon through cAMP-CAPK-mediated inhibition. This explains why when grown with glucose E. coli will lose the ability to internalize AI-2 (because of catabolite repression). When grown normally, AI-2 presence is transient.
A first X-ray structure of a receptor (LuxP) was discovered in Vibrio harveyi in 2002, together with its inducer (AI-2), which is one of the few biomolecules containing boron (Nature 415, 545ff PDF). Autoinducer-2 is conserved among many bacterial species, including Escherichia coli, an enteric bacterium and model organism for Gram-negative bacteria. Autoinducer-2 appears to be used for interspecies communication because of this conservation.
References
Citations
- ↑ Camilli A and Bassler BL (2006). Bacterial small-molecule signaling pathways. Science 311: 1113-1116.
- ↑ Pradeep K. Singh, Amy L. Schaefer, Matthew R. Parsek,Thomas O. Moningerk, Michael J. Welsh, & E. P. Greenberg. (2000) Quorum-sensing signals indicate that cystic fibrosis lungs are infected with bacterial biofilms NATURE VOL 407 12 OCTOBER 2000 page 762-762.
- ↑ Keller, Laurent and Surette, Michael G. (2006) Communication in bacteria: an ecological and evolutionary perspective. Nature Reviews Microbiology 4, 249-258 (April 2006) | doi:10.1038/nrmicro1383
- ↑ Camilli A and Bassler BL (2006). Bacterial small-molecule signaling pathways. Science 311: 1113-1116.
- ↑ Waters CM and Bassler BL (2005). Quorum sensing: cell-to-cell communication in bacteria. Annu Rev Cell Dev Biol 21: 319-346.
- ↑ Shimkets, L. J. (1999) Intercellular signaling during fruiting-body development of Myxococcus xanthus. Annu. Rev. Microbiol. 53, pages 525–549.
- ↑ Kruse, T. , Lobedanz, S. , Berthelsen, N. M. & Sogaard-Andersen, L. (2001) C-signal: a cell surface-associated morphogen that induces and co-ordinates multicellular fruiting body morphogenesis and sporulation in Myxococcus xanthus. Mol. Microbiol. 40, pages 156–168 .
- ↑ Tom Burr, Anne M. L. Barnard, Mark J. Corbett, Clare L. Pemberton, Natalie J. L. Simpson and George P. C. Salmond (2006) Identification of the central quorum sensing regulator of virulence in the enteric phytopathogen, Erwinia carotovora: the VirR repressor. Molecular Microbiology Volume 59 Issue 1 Page 113 - January 2006 doi:10.1111/j.1365-2958.2005.04939.x Volume 59 Issue 1
- ↑ Solomon, J. M. , Lazazzera, B. A. & Grossman, A. D.(1996) Purification and characterization of an extracellular peptide factor that affects two different developmental pathways in Bacillus subtilis. Genes Dev. 10, pages 2014–2024.
- ↑ Shompole, S. et al.(2003) Biphasic intracellular expression of Staphylococcus aureus virulence factors and evidence for Agr-mediated diffusion sensing. Mol. Microbiol. 49, 919–927
Further reading
- Banin, E., M. L. Vasil, and E. P. Greenberg (2005). Iron and Pseudomonas aeruginosa biofilm formation. Proc Natl Acad Sci U S A 102: 11076-81.
- Bassler BL (2006). Cell-to-cell communication in bacteria: a chemical discourse. Harvey Lect. 2004-2005 100: 123-142.
- Bassler BL and Losick R (2006). Bacterially speaking. Cell 125: 237-246.
- Camilli A and Bassler BL (2006). Bacterial small-molecule signaling pathways. Science 311: 1113-1116.
- Kolter, R. and E. P. Greenberg (2006). Microbial sciences: the superficial life of microbes. Nature 441: 300-2.
- Waters CM and Bassler BL (2005). Quorum sensing: cell-to-cell communication in bacteria. Annu Rev Cell Dev Biol 21: 319-346.
- Waters CM, Bassler BL (2006). The Vibrio harveyi quorum-sensing system uses shared regulatory components to discriminate between multiple autoinducers. Genes Dev 20: 2754-2767.
- Xavier KB and Bassler BL (2005). Regulation of uptake and processing of the quorum-sensing autoinducer AI-2 in Escherichia coli. J Bacteriol 187: 238-248.
- Xavier KB and Bassler BL (2005). Interference with AI-2-mediated bacterial cell-cell communication. Nature 437: 750-753.